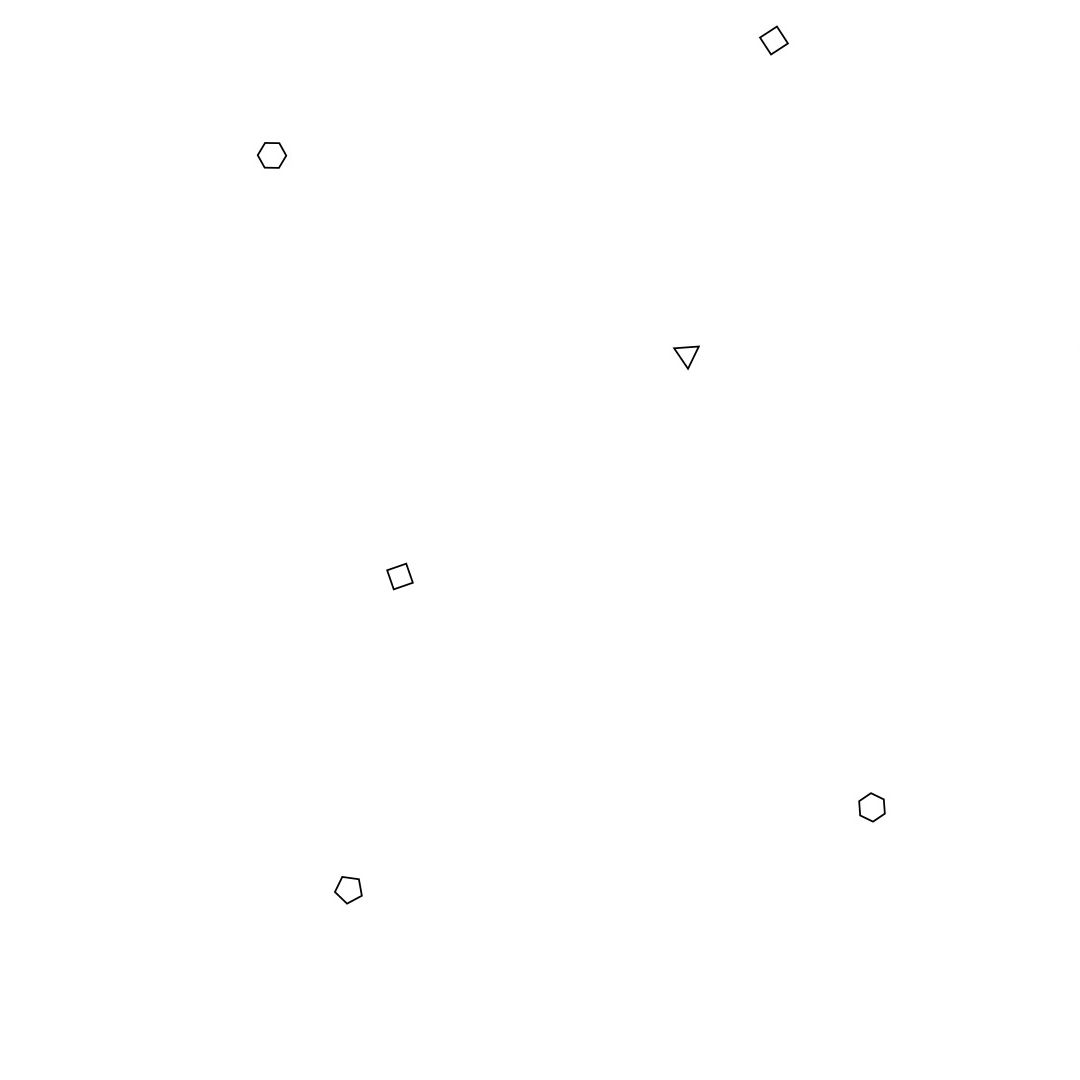
Research Projects
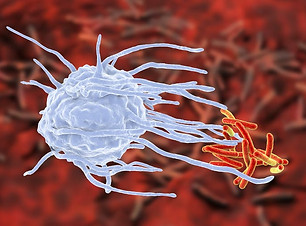
Macrophage
10/2022 - present
Macrophage response mechanism could extend an insight molecular knowledge into human immunity. We are finding the bacterial protein activating the human innate immunity.
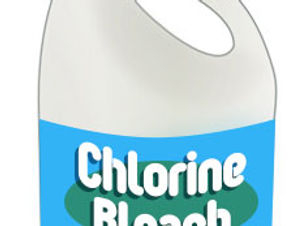
BIOCIDES
01/2019 - 012/2019; continuing
Bleach in hospitals and household induces bacterial response against antibiotics and biocides resistance. Mechanisms are still under investigation and extending for biosensor develop.
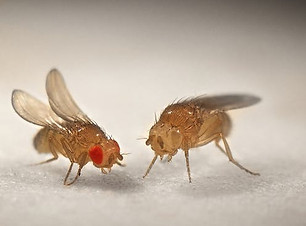
Drosophila
03/2021 - present
Drosophila melanogaster was used as the model host system for bacterial infections. Fruit fly response mechanism could extend an insight molecular knowledge.
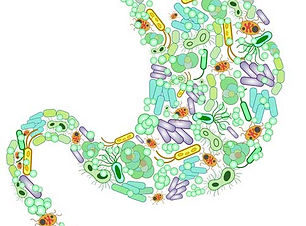
MICROBIOME
10/2018 - present
Microbiome is our new path in collaborate with Betagro company: A comparative metagenomic analysis platform for the robust discovery of beneficial microbiota.
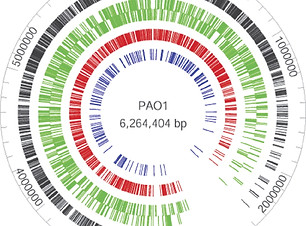
PAE GENETICS
10/2018 - present
Pseudomonas aeruginosa genomic library will be constructed and modified to identify novel genes in virulence network and antimicrobial resistance cassettes.

Antimicrobial testing
10/2021 - present
Several products are tested for antimicrobial activtity testing from our laboratory
Research Areas
> Bacteriology research is diverse and is directed towards drug resistant pathogens (e.g. Pseudomonas aeruginosa) and plant pathogenic bacteria (e.g. Xanthomonas campestris) and emerging pathogens or bacteria of biodefense importance (e.g. Burkholderia pseudomallei). Efforts include studying antibacterial resistance mechanisms, identification of novel drug targets, drug discovery and development, immunotherapy, bacterial physiology, mechanisms of pathogenesis, development of animal models for emerging infectious diseases and vaccine discovery. These studies are facilitated by state-of-the-art BSL2 laboratory facilities. Many of these are located in the EBI center at SCMU and the Biotech lab at CRI.
>> Human pathogens >>> Pseudomonas aeruginosa
​
​
>> Plant pathogens >>> Xanthomonas campestris
Xanthomonas campestris (Xcc) is a soil-borne plant pathogenic bacterium. It causes black-rot disease in a large number of species of Brassicaceae worldwide. The plants include economically important vegetable Brassica crops and a number of other cruciferous crops such as cabbages and ornamental crucifers, and weeds. Typical symptoms of black rod disease are V-shaped yellow lesions starting from the leaf margins and blackening of the veins. The symptoms of black rot are occurred shown in Figure 2.1A. With this mechanism of infection, the virulence of Xcc strains has been determined by leaf-clipping method in Chinese radish (Raphanus sativus) plants (Dow, et al., 1994) as shown in Figure 2.1B. Spraying healthy plants with copper fungicides may reduce the spread of the bacteria in the field. However, once the plants have been infected, Xcc will eventually spread to the seed stalk and inhibit the growth of a healthy offspring.

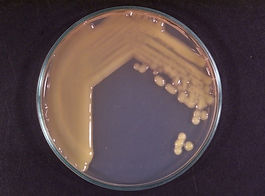
P. aeruginosa is one of the top three causes of opportunistic human infections, particularly in cystic fibrosis, cancer and acquired immune-deficiency syndrome (AIDS) patients with compromised host defense mechanisms. P. aeruginosa is widespread around the world and has no specific epidemic distribution. Moreover, P. aeruginosa-infected outbreaks were recently reported in all age range. It has been claimed as a cause in outbreaks of many syndromes during the past year such as a contact-lens infection in Thailand, 2011 and a hot-foot syndrome in Germany, 2012. The intrinsic and emerging antibiotic resistance confounds the ability to eradicate or control P. aeruginosa infections. Currently, the World Health Organization has listed P. aeruginosa in their critical priority list for drug development due to the emergence of multi-drug and pan-drug resistance isolates.
P. aeruginosa are often existing even in the hospital. Therefore, hospitalized people are also in high risk to get infections. The bacteria can spread through food, medical tools and any solution used in the hospitals. It is one of the most common pathogenic bacteria, which are isolated from patients who are hospitalized more than a week. An example of this outbreak, in 2005, Brazilian model was dead by the bacterial infection in the hospital after she occasionally got her leg-operation. Because this bacterium has developed many protective mechanisms or adapted to live in harsh conditions for example weak antiseptics, high temperature, high salts, and even antibiotics. It is often observed growing in tapped water due to its very simple nutritional requirements. Therefore, it can contaminate in any place, live in any environment, and infect anyone in a certain time. Pseudomonas infections are more complicated and life threatening.
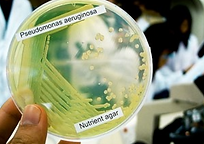
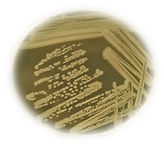
Oxidative stress
In general, aerobic bacteria always come into contact with reactive oxygen species (ROS) which derived from both internal and external sources. Oxidative stress is defined as an imbalance between the production and the elimination of reactive oxygen species (ROS), leading to an excess of pro-oxidants inside the cell, resulting from cellular metabolism and from exogenous sources such as redox-cycling drugs and ionizing radiations. Aerobic metabolism is an important source of internal ROS production. Oxygen itself is often the source of such radicals, as arising from part of a reduction in partially normal metabolism, and some of ROS can run off. The O2 accepts single electron from components of the cellular electron transport chain resulting in the production of the radical superoxide (O2-), the non-radical hydrogen peroxide (H2O2) and the hydroxyl radical (OH·). The biochemical pathways can also give rise to harmful oxidants. For example, the process of denitrification leads to the unwanted formation of nitric oxide (NO·) in the presence of superoxide, spontaneously converts to peroxynitrite (ONOO·)
Virulence​
The pathogenesis of P. aeruginosa infection is multifactorial and complex which requires many factors. P. aeruginosa possesses many cell components and cellular products that responsible to virulence such as adhesions, exotoxins, proteases, and a type III secretion system. To colonize on the host tissue, P. aeruginosa requires both fimbrial and non-fimbrial adhesions. For fimbrial adhesins, P. aeruginosa has type IV pili, which promote the adherence by binding to host cell glycoproteins and low molecular weight polypeptides. P. aeruginosa also has three sets of type I fimbriae, which enhance the adherence to abiotic surface and in biofilm formation. Non-fimbrial adhesins, such as flagella, outer membrane proteins, lipopolysaccharide, and alginate also encourage P. aeruginosa adherence. After attach to host cells, it will release many extracellular products to facilitate tissue invasion.
Exotoxin A is the most potent of the P. aeruginosa exotoxins and is produced by most clinical isolates. In bacterial pathogenesis, exotoxin A is responsible for the local tissue damages, bacterial invasion, and possibly immunosuppression within the eukaryotic host. Exotoxin A can inhibit protein synthesis by catalyse the ADP-ribosylation of eukaryotic elongation factor-2, which inactivate this enzyme and cause cell death. Protease and phospholipase are another virulence factors secreted by this bacterium. P. aeruginosa produces many enzymes to facilitate tissue invasion including elastase, alkaline proteases, hemolysin (phospholipase and lecithinase), cytotoxin (leukocidin), siderophores, and pyocyanin pigment. Elastase and alkaline protease degrade a broad range of host substrates such as, laminin, fibrinogen, collagen, transferring, complement components, immunoglobulin resulted in inactivation of host defence proteins. Elastase can also degrade surfactant proteins A and D, which have an important role in innate immune response. Phospholipase and lecithinase act together to break down lipids and lecithin. Leukocidin is a pore-forming protein that has cytotoxic effect to host cells.
To inject toxin into host cells, P. aeruginosa encodes type-III secretion system which are common to many Gram-negative pathogens. Type-III secretion systems compose of three components: the secretion apparatus, the translocation, and the secreted toxins (effector proteins). Four secreted toxins, ExoS, ExoT, ExoU, and ExoY act together to inhibit phagocytosis, promote tissue destruction, and impair wound healing. In addition to type-III secretion system, P. aeruginosa develops mechanisms to control expression of genes involved in adaptaion to environment called quorum sensing (cell-to-cell signaling). This signaling mechanism helps P. aeruginosa to regulate genes in a density-dependent manner and thought to function by allowing P. aeruginosa to reach critical population density before starting quorum sensing and producing virulence factors. By delaying the production of virulence factors, P. aeruginosa can avoid an immune response from host until have enough bacterial cells to overcome the host.
Iron-sulfur cluster
Iron-sulfur cluster, [Fe-S], proteins are required for essential functions of many biological processes across all domains of life due to the abandon of iron and sulfur on the earth crush and their structural versatility and chemical reactivity. Over 150 and 50 proteins from total identified proteins in Escherichia coli and Mycobacterium tuberculosis, respectively, contain [Fe-S] centers, which can act as catalysts or redox sensor. These [Fe-S] proteins have prominent roles in multiple important biological processes, including biosynthetic pathways, respiration, central metabolism, photosynthesis, nitrogen fixation, DNA repair, RNA modification, and gene regulation. A bacteria-specific ferredoxin (encoded from fdx1, PA0362) was proposed as an example of [Fe-S] proteins that are essential in Pseudomonas aeruginosa. The most common type of [Fe-S] centers is approximately 90% cubic [4Fe-4S] type, others are rhombic [2Fe-2S], [3Fe-4S], [8Fe-7S] and other types. In most of the [Fe-S] proteins, cysteine residues coordinate the iron ions of the [Fe-S]; however, histidinyl residues can be required for cluster ligation. This notable exceptions are the Rieske proteins, which have [2Fe-2S] clusters featuring (Cys)2(His)2 ligation and the E. coli transcriptional regulator IscR and Clostridium acetobutylicum [FeFe]-hydrogenase H cluster maturation scaffold HydF, which contain [2Fe-2S] clusters featuring (Cys)3(His)1 ligation.
One of the primary roles of Fe-S biogenesis regulation is to maintain, rebuild, and possibly repair the Fe-S cluster-containing proteins in different environmental conditions. In E. coli, the regulator IscR is responsible as the core of Fe-S homeostasis regulation but additional regulators are involved including Fur and OxyR. Our laboratory has shown the regulatory mechanisms of P. aeruginosa IscR in order to regulate several genes in response to oxidative stress conditions. These genes under IscR regulon are described such as thiol-peroxidase, isc-operon, and others.
Antibiotic resistance
The planktonic bacteria are killed by antibiotic treatment, but the biofilm bacteria survive and endure for many years. P. aeruginosa causes acute diseases owing to toxin-producing planktonic cells; it can cause chronic diseases because of slime-protected biofilms, and can switch between these two to cause diseases with acute and chronic components. Therefore, the ability to form biofilm of P. aeruginosa is an important factor for infection into host and resistance against antibiotic. Moreover, the size and complexity of the P. aeruginosa genome affect an evolutionary adaptation to grow in diverse environments and resist a variety of antimicrobial substances.
Fluoroquinolone is derivatives of 4-quinolones that are the core structure of the quinolone antibiotic. The fluoroquinolones are antibacterial agents that target two essential bacterial enzymes, DNA gyrase or topoisomerase II and topoisomerase IV that can cuts both strands of DNA double helix and rejoins the cut strand. Gyrase controls DNA supercoiling and relaxs topological stress that originating from the translocation of transcription and replication. Moreover, topoisomerase IV resolves interlinked daughter chromosomes following DNA replication in order to segregate into daughter cells. However, the fluoroquinolone do not eliminate topoisomerase function but trap at the molecule of gyrase and topoisomerase IV on DNA replication. Therefore, double-strand DNA break cannot rejoin and leads to the lethal of bacteria from double-strand DNA breaks poisons.
Our laboratory has proposed that NfuA functions in Fe-S cluster biogenesis and involves in fluoroquinilone resistance in P. aeruginosa. Deletion of nfuA gene resulted in an increased susceptibility against antibiotic drugs in this group. We showed the mechanism of NfuA related to these drugs was resulted from the oxiative DNA damages. Recently, we has shown that deletion of grxD in P. aeruginosa caused a decreased resistance against polymyxins. However, its mechanism was not related to an oxidative stress as we proposed, we are under investigating the side mechanisms of GrxD.
Protein repair
During the oxidative stress, protein oxidation is one of the most important processes for bacterial survival. Many amino acids within the protein can be modified resulting in oxidatively modified proteins that can lead to the secondary unfolding of proteins and modification by non-protein oxidation products. This oxidative attack on proteins also results in site-specific amino acid modifications, fragmentation of the polypeptide chain, aggregation and cross-linking, altered electrical charge and surface properties, and changed susceptibility to proteolysis. If high concentrations of oxidized proteins inside the cell might have adverse effects, the worst outcome can be cell death. Indeed, the cell needs some mechanisms to degrade these toxic oxidized proteins such as protein repair or protein degradation. These processes are more importance because several diseases, like Alzheimer’s disease, Parkinson’s disease, arteriosclerosis, and the cell-aging process, are involved in the accumulation of oxidized proteins.
Broadly various categories of the oxidants can attack the protein into the backbone, side-chain sites, or multiple sites. Unlike the non-radicals, the radicals can react rapidly with the backbone of proteins and generate carbon-centered radical as the final product. Another reaction with radical will expected to be a limited process in most situations due to the low radical flux encountered in most biological systems and reaction with O2 to give a peroxyl radical. The peroxyl radical will give rise to backbone fragmentation or undergo an alternative product to the imine pathway. Another oxidative target site of the oxidants is amino acid side-chain. There are at least 20 different types of amino acid side-chains result in a numerous variety of potential reaction sites and products on reaction with oxidants as only 4 main categories, which are aliphatic, aromatic, Cys and cystine residues, and Methionine.
Gene regulation​
During process of infection, bacteria are exposed to exogenous oxidants generated by phagocytic cell. Bacteria have an ability to survive in the oxidative stress by a broad variety of mechanism to protect and repair the damage caused by ROS. Several antioxidant enzymes is responsible for degrading ROS prior damage occurrence such as catalases (Kat), superoxide dismutases (SOD), peroxiredoxins (Prxs), alkyl hydroperoxide reductase (Ahp), organic hydroperoxide resistance (Ohr), and thiol peroxidase (Tpx).
To control these gene expression efficiently, bacteria have several major regulators activated during oxidative stress and acted as oxidant sensing, including OxyR, SoxR, OhrR, OspR, Fur, HypT, and MosR, have been characterized. These regulators were responded in different manners and mechanisms in which our laboratory is under investigating.
Fur, ferric uptake regulator, is a transcriptional regulator that important for maintenance of iron homeostasis in many bacteria. Fur is a homodimeric metalloprotein, in which after forming complex with iron, can regulate the transcription of genes by binding to a Fur box in the promoter region. Fur usually mediates bacterial iron-responsive gene regulation. It acts as an iron-dependent positive regulator of katA transcription in S. aureus. Hence, fur mutants show reduction in catalase activity as well as increasing in sensitivity to peroxide stress.
OhrR, a member of MarR family, is an organic peroxide-inducible transcription repressor. In the absence of organic peroxides, OhrR functions as a dimeric repressor by binding to the ohr promoter region. Organic hydroperoxide resistance (Ohr) thiol-dependent peroxidases are important enzymes to detoxify organic hydroperoxides in many bacteria including Xanthomonas. B. subtilis OhrR contains only one cysteine while X. campestris OhrR contains a second cysteine located on the COOH terminal of the OhrR protein (C22 and C127). Therefore, oxidation of OhrR results in cross-linking of two OhrR monomers which leading to the inactivation of its repressor function.